ABSTRACT
CRISPR-cas gene editing in crop plants could significantly speed up the progress of breeding programs. Strikingly in the agricultural sector, the number of CRISPR-cas patents originating from Europe trails far behind the USA and China. Examining field trials is another mean to compile biotechnological innovation in plant breeding. We examined field trials since 2002 and more recently from 2015 to 2020 with the emergence of CRISPR-cas in plant breeding. A total of 881 field trials were conducted in the EU from 2002 to June 2020 and maize represents 54.3% of them. Disparities exist within the EU Member states and Spain leads the EU field trials with almost half of them. The drop of field trials in the EU since 2006 can be linked to strict GMO regulations. From January 2015 to June 2020, only 48 field trials were conducted, or are in progress, in eight countries compared to the 19 countries between 2002 and 2015. Spain and Sweden are ranking first with 28.3% of these field trials, while the UK is holding the third place with 17%. Only 5 field trials use CRISPR-Cas9. Agronomic improvement comes first, followed by nutritional enhancement and biotic stress resistance as traits of field trials. Regarding the biotech crops, potato is the most tested crop with a fifth of the field trials (20.8%). The implications of regulatory policy in the restrictive deployment of NBTs for plant improvement in Europe are discussed as well as the need for a new regulation.
INTRODUCTION
Limited genetic diversity restricts the amount of crop improvement that can be achieved through conventional breeding approaches. Next-generation sequencing studies demonstrate that across their genomes, populations of plant species typically carry millions of single nucleotide polymorphisms and many copy number and structural variants. De novo (spontaneous) mutations occur at rates of approximately 10-8 to 10-9 per site per generation, while variation induced by chemical treatment or ionizing radiation results in higher mutation rates. The new breeding techniques (NBTs) using site-directed nucleases (SDNs) can accelerate crop improvement through the introduction of genetic variation in a targeted manner. SDNs used for targeted genome editing are powerful new tools to introduce precise genetic changes into plants since 2002. Like traditional approaches, such as conventional crossing and induced mutagenesis, genome editing aims to improve crop.
The convergence of low-cost genome sequencing with improved computational power and high throughput phenotyping technologies has accelerated the identification of genes underlying important agronomic traits relevant to food production and quality. Together with the advancement of whole genome sequencing technologies, NBTs have rapidly emerged in recent years. Availability of whole genome sequence information for number of crops along with the advancement in genome-editing techniques provides several possibilities to achieve desirable traits.
Compared to the early versions of SDNs of NBTs, such as oligonucleotide-directed mutagenesis (ODM), meganucleases (MNs), zinc fingers nucleases (ZFNs), and transcription activator-like effector nucleases (TALENs), the clustered regularly interspaced short palindromic repeat (CRISPR)-system is capable of altering a genome more efficiently and with high accuracy. The CRISPR-cas is a rapidly growing genome editing technique being effectively applied in different plant organisms, that includes both model and crop plants (Ricroch, Clairand & Harwood 2017; Pfeiffer, Quétier & Ricroch A. 2018). In recent times, CRISPR-cas gene editing is being considered as a technology which revolutionized fundamental as well as applied research in plant breeding since 2014 and initiated on a wheat (Wang et al. 2014). Meanwhile genome editing using a CRISPR-cas has been successfully demonstrated in many cereal crops including barley, maize and rice. These NBTs could drive the development of traits in new crops and allow also biofortification, yield improvements, and pest and disease resistance, adaptation to climate change, and industrial and pharmaceutical applications (Ricroch 2019). A recent innovation concerns the optimization of nitrogen nutrition or water use efficiency. The development of climate-resilient crop varieties able to maintain yield and quality is essential to food security and economic returns.
Europe played a major role in plant biotechnology with pioneering discoveries on transgenesis by Van Montagu in Belgium and Schell in Germany (see Herrera-Estrella et al. 1983). In this present study, we examine the place of Europe in the NBTs landscape for agricultural innovation. Since repetitive bans of cultivation of transgenic crops, we are examining whether the NBTs are inducing a new dynamic in plant breeding programs in Europe. we compiled field trials of biotechnological plants since 2002 to follow their evolution, and particularly since 2015 with the availability of NBTs and the implementation of the EU Directive 2015/412 (the so-called ‘opt-out’ Directive).
1. FIELD TRIALS IN EU FROM 2002 TO 2020
In 2019, we performed an exhaustive compilation of patents involving a CRISPR-cas from 2012 to 2018 (Martin-Laffon, Kuntz and Ricroch 2019). This landscape showed that laboratories in the USA played a pioneer role in the original invention. Patents using CRISPR-cas in relation to plants appeared since September 2012 (Dow Agrosciences/Sangamo Biosciences, USA). A total of 262 patents were deposited with claims related to plants. Some other patents, without direct agricultural application, such as the use of plants for molecule production, or specific metabolic changes, or as a research model (Arabidopsis, barley and rice) were grouped in this plant category. China is leading the plant category (60.5%) followed by the USA (26%) while patents from European origin represent only 8% (17 patents of which Germany and Netherlands contributed to 6 and 5 patents, respectively). Japan and Korea represent 2.3% each (5 patents). Rice is the most represented plant followed by maize and wheat. This can be explained by the massive investment in biotechnology in China (Ricroch 2020 in press). An example in the agricultural field of a technical incentive for China’s investment could be the national importance of rice cultivation in a context of climate change and related biotic constraints. Private companies with the most deposits are Dupont Pioneer (USA), KWS Saat (Germany), Keygene (Netherlands), Dow Agrosciences (USA), Beijing DBN Technology (China). Public depositors are mostly represented by Chinese public organizations. University of California is the most important American depositor for agricultural sector. Private companies filed 27% of these patents (USA, China, Netherlands and Germany). Public depositors are mostly represented by Chinese research organizations. The number of CRISPR-cas patents originating from Europe trails far behind China and USA. Claims relate to plant breeding, technical improvement of the use of CRISPR-cas, use of plant model, research, virus resistance, epigenome editing and molecule production.
As Europe plays a minor role in the patent landscape, we gathered field trials carried out in Europe to examine the breeding programs since 2002 and more recently (2015-2020) as CRISPR-cas is a new tool used in breeding programs However since March 2015 member states have the possibility to adopt legally binding acts restricting or prohibiting the cultivation of GMOs in their territory according to the EU Directive 2015/412. The website of the European Commission (EC) provides field trials in the EU since 2002 (https://gmoinfo.jrc.ec.europa.eu/gmp_browse.aspx). A total of 881 field trials were carried out in Europe from 2002 to June 2020.
The success of a breeding program depends on its adequate positioning within the set of innovations adopted by breeders. In Europe EuropaBio (2020) counts 23 EU-funded projects on CRISPR applications in agriculture (retrieved in mid-2019). The EU appears to have invested more than 34 million EUR (in these projects as of mid-2019) such as implementation of CRISPR-Cas9 technology in melon to edit fruit ripening and CMV resistant genes or for improved resistance to abiotic stress in cereals for examples.
Field trials have to be conducted under conditions which represent the real agronomic practices under which the plants are expected to be grown commercially. In 2019 a little over 3500 new plant varieties were applied at the Community Plant Variety Office (a European Union agency, which manages the European Union system of plant variety rights covering the 27 Member States). That is almost 10 new plant varieties per day. As Europe plays a minor role in the patent landscape, we gathered field trials carried out in Europe to examine the breeding programs as innovation. In order to understand possible trends in R&D, we gathered all the field trials conducted in the EU since 2002 using transgenesis and since 2005 using transgenesis and NBTs. These European data help us to discuss the evolution of the research since the emergence of NBTs and highlight the particularities of the EU.
Many breeding programs have been implemented in Europe from 2002 with a maximum of 124 field trials in 2006. Strict regulations led to the drop of field trials in the EU since 2006 as seen in figure1.

Fig. 1: Number of field trials in the EU per year (2002-2020) (date of acknowledgement from the Member State Competent Authority) (total: 881 field trials)
First of all, taking into account the 881 field trials conducted in the EU from 2002 to June 2020 and approved by the EC, the maize crop is far ahead from the other crops with 54.3% of the field trials. Moreover, Spain leads the EU field trials with almost half of them followed by France, Germany and Sweden (figure 2).
Fig. 2: Distribution of the field trials according to countries and crops in the EU (2002-2020) (total: 881 field trials)
a. Member states (19) conducting field trials.

b. Crop species (36) used in field trials.
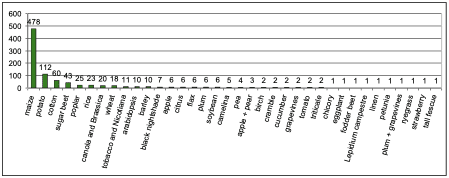
Looking at traits tested in field for agronomic evaluation we noticed that breeders develop new plant varieties that make it easier for the farmer to harvest the crops (conservation tillage in sugarbeet, gene flow control in red rice, flax) or have
- a higher yield (modified morphology in cucumber, production of recombinant spider silk in potato as bioreactor; nitrogen efficiency, grain filling, improved photosynthesis, flowering earliness in maize; modified plant architecture, flowering and fruiting behaviour in citrange, grain production in camelina, rice),
- biotic stress resistance (more resistance against pests and diseases in apple, cotton, cucumber, grapevine, lemon, maize, oilseed rape, orange, plum, potato, rice, sugarbeet, wheat and more herbicide resistance in cotton, maize, oilseed rape, sugarbeet, soybean, wheat),
- abiotic stress resistance (better drought tolerance in cotton, maize, pea, potato, wood),
- biofortification and nutritional improvement (altered starch composition in potato, maize, more vitamins, higher levels of beneficial compounds such as altered oil content in oilseed rape, camelina and altered industrial oil content in crambe, elevated anthocyanin levels in apple, more phytase in barley, altered sulphur metabolism in Brassica oleracea),
- biomass production properties in fescue, maize, poplar and tobacco,
- pharmaceutical traits in barley, potato, maize,
- breeding traits (parthenocarping processing in tomato, strawberry; hybrids aspens; developed energy crops for bioethanol production and Lepidium campestre as a new and cover oil crop).
2. FIELD TRIALS IN EU FROM 2015 TO2020
Is this trend changing with the use of NBTs? We focus on all field trials carried since 2015 or currently in progress in the EU as the EU Directive 2015/412 is implemented and as CRISPR-cas emerges as a new tool used in breeding programs (figure 3). Indeed breeders can implement new technologies and tools to accelerate genetic gain in breeding programs such as the use of CRISPR-Cas9.
Data was extracted from the European Commission website (https://gmoinfo.jrc.ec.europa.eu/gmp_browse.aspx).
From January 2015 to June 2020, a few field trials (48) were conducted or in progress. Eight countries only are still carrying out field trials between 2015 and 2020 compared to the 19 countries between 2002 and 2015. In figure 3 Spain and Sweden are ranking first with 28.3% of the field trials each one (2015-2020). The UK, which will maybe be removed from the Member states list, is holding the third place with 17%. Coming next, Belgium and Czech Republic have conducted respectively 9.4% and 7.5% of the field trials. Finally, two field trials start in the Netherlands, one in Poland and one in Finland. The two most populated European countries (France and Germany) carrying out a lot of trials between 2002 and 2015 do not implement any of them during the period 2015-2020.
Between 2015 and 2020, 11 countries have renounced field trials (Denmark, France, Germany, Hungary, Iceland, Ireland, Italy, Lithuania, Portugal, and Slovakia).
Fig. 3: Distribution of the field trials according to countries and crops in the EU (2015-2020) (total: 48 field trials)
a. Member states (8) conducting field trials.

b. Crop species (16) used in field trials.
Hybrid aspen and grey poplar were considered both as ‘Poplar’.

Five private companies only among the 23 institutes (table 1) are carrying out field trials between 2015 and 2020 (IDEN Biotechnology and Biomass Booster SL in Spain, Lyckeby Starch AB, SweTree Technologies AB and Syngenta in Sweden, Usovsko a.s. in Czech Republic). Mainly public researchand medium companies (apart the big compagny Syngenta) are carrying out field trials.
Table 1: Distribution of the field trials according to institutes in the EU (2015-2020) (total: 48 field trials)
Institute | Number of field trials |
Swedish University of Agricultural Sciences,Sweden | 8 |
John Innes Centre,UK | 5 |
IDEN Biotechnology,Spain | 4 |
Rothamsted Research,UK | 4 |
VIB (Vlaams Interuniversitair Instituut voor Biotechnologie), Belgium | 4 |
University of Lleida,Spain | 3 |
Biomass Booster, SL,Spain | 2 |
Centro Nacional de Biotecnología – CSIC,Spain | 2 |
Universidad Pública de Navarra – CSIC,Spain | 2 |
Agencia Estatal Consejo Superior de Investigaciones Cientificas,Spain | 1 |
Centro de Edafología y Biología Aplicada del Segura,Spain | 1 |
Crop Research Institute,Czech Republic | 1 |
Fruit Research and Development Station Bistrita,Romania | 1 |
Institute of Experimental Botany AS CR,Czech Republic | 1 |
Lyckeby Starch AB,Sweden | 1 |
Palacky University in Olomouc,Czech Republic | 1 |
Wageningen University,Netherlands | 1 |
Sveriges LantbruksuniversitetSweden | 1 |
SweTree Technologies AB,Sweden | 1 |
Syngenta Seeds AB,Sweden | 1 |
University of Helsinki,Finland | 1 |
Usovsko a.s.,Czech Republic | 1 |
Umea University,Sweden | 1 |
Warsaw University of Life Sciences,Poland | 1 |
The evolution in the type of crop tested in field conditions since 2015 can be explained by the emergence of NBTs. Regarding the biotech crops, potato is the most tested crop with a fifth of the field trials (20.8%). Maize ranked second followed by aspen, wheat, Camelina and plum. Thus, two trials were conducted on apple, canola and rice. Peper grass is the only one new crop tested for increased oleic acid content) in Sweden.
Transgenic plants are still tested in field trials. Using CRISPR-cas9 VIB (Vlaams Interuniversitair Instituut voor Biotechnologie) is the first institute to develop an abiotic biosensor (signal of environmental abiotic stress at DNA level) in maize tested in a field trial in 2018. Genetic modification between 2015 to 2020is still provided by the conventional transgenesis with 84.9% of trials (Agrobacterium tumefaciens (79.2%) or biolistic used in rice, maize and soybean (5.7%)) but more and more crops in field trials are genetically engineered with CRISPR-Cas (9.4 %), modified with RNAi technique (3.8%) or both (RNAi+CRISPR-Cas9 (1.9%)). RNA interference (RNAi) is a biological process allowing plants to transfer small regulatory RNAs to invading pathogens to trigger the silencing of target virulence genes. There is a growing proportion of NBTs in the EU field trials leading to a new generation of varieties. Indeed, 5 field trials rely on CRISPR-Cas9 (in 2019 potato with less amylose starch at Lyckeby Starch AB in Sweden, tobacco with delayed flowering at Agencia Estatal Consejo Superior de Investigaciones Cientificas in Spain, Brassica oleracea regulating sulfur metabolism at the John Innes Centre in UK, and in 2018 maize with abiotic biosensor (2 trials) at VIB in Belgium) and in 2017 two field trials rely on RNAi technique (plum with pox virus resistance at the Crop Research Institute in Czech Republic and potato with late blight resistance at the John Innes Centre in UK). In 2019 one Camelina variety with modified oil was designed by both RNAi and CRISPR-Cas9 at the Rothamsted Research in UK.
We examined the evolution of the distribution of traits in terms of applications in EU field trials from 2015 to 2020 with the emergence of CRISPR-Cas9 (figure 4). Agronomic improvement comes first followed by nutritional enhancement and biotic stress resistance. Then, the biotech plants with industrial applications are also well-represented due to various field trials based on trees (poplar and cramble) in order to meet wood and paper industries demands. Five field trials concern resistance to abiotic stresses. Crop plants were designed to address several global challenges. Thus, transgenic barley, rice and soybean can be classified as biotech crops with therapeutic application.

Fig. 4: Traits of biotech plants in field trials in the EU (2015-2020) (total: 48 field trials)
Table 2: Distribution of the field trials according to traits in the EU (2015-2020)
(total: 48 field trials)
Trait | Number of field trials |
Oil quality | 8 |
Fungal disease resistance | 4 |
Pathogen resistance | 3 |
Abiotic biosensor | 2 |
Biomass production enhanced | 2 |
Drought tolerance | 2 |
Growth characteristic enhanced | 2 |
Increased starch content | 2 |
Increased yield | 2 |
Increased the rooting ability | 2 |
Sucrose synthase overexpressed | 2 |
Wood biomass increased | 2 |
Anthocyanin levels increased | 1 |
Biofortification of vitamins (A, B, C) | 1 |
Vitamin A production + Pest resistance (Bt) | 1 |
Biomass production enhanced | 1 |
Delayed flowering | 1 |
Glyphosate herbicide tolerance | 1 |
Hight iron content | 1 |
Less of amylose starch | 1 |
Regulating sulphur metabolism | |
Photosynthesis efficiency increased | 1 |
Root system enhanced | 1 |
Rootstocks abilities for peach and apricot | 1 |
Accumulation of 3 molecules anti-VIH | 1 |
Cathelicidin peptide LL-37 – Human antimicrobial peptide | 1 |
Recombinant LTB protein (Human tumor necrosis factor C ) | 1 |
CONCLUSION
The recent global climate change has directly impacted major biotic and abiotic stress factors affecting crop productivity worldwide. Therefore, the need is to develop sustainable multiple stress tolerant crops. NBTs and the modern biotechnological approaches can cope with climate change faster than conventional tools. NBTs could also be used for developing crops with novel agro-traits that can help reduce pesticide, fertilizer and water and nitrogen use and improve food quality and safety. New crop varieties combine high yield potential coupled with several genetic traits. The classification of CRISPR-type gene editing patents worldwide in plants shows an unexpected switch in the balance of forces in favour of China while providing no indication that Europe, which has lost the “GMO” battle, is in a position of regaining forces in this new biotech battlefield.
On 25 July 2018, the European Court of Justice (CJEU) ruled on the interpretation of the definition of the term “genetically modified organism” in the GMO Directive 2001/18/EC. It follows from the ruling that all organisms produced by genome editing are subject to the legal framework applicable to release, placing on the market, labelling, and traceability of GMOs. France is implementing the CJEU ruling (C-528/16) on mutagenesis procedures on June 2020. Plants resulting from in vitro mutagenesis procedures constitute GMOs and are subject to genetic engineering and environmental protection legislation. The European Commission can implement the ECJ ruling for the EU and the Council of State (Conseil d’État), French highest administrative court, must implement it for France. The French Council of State rendered its judgement on 07.02.2020. The court largely followed the CJEU ruling.
Gene editing products would seem to follow a much faster development rate from bench to market than GM products if the regulation is not too stringent. Today few plants developed with NBTs are in the market worldwide. In Europe Regulatory constraints, including environment and health impact assessments of transgenic plants, have delayed approvals and increased costs for transgenic plants (Ricroch, Boisron & Kuntz 2015). While the science is exciting and some clear benefits are already observable, overregulation and public misperceptions may obstruct efficient development and use of NBTs (Quaim 2020). Our present study shows that overregulation has slowed down innovation in Europe as very few field trials tests using CRISPR have been set up and patents deposited. The problem could be attributed to a hostile cultural (“precautionary”) climate against innovations in Europe, including biotechnology (Kuntz & Berezow 2017). It would be a delusion not to consider that the successive GMO bans in Europe have not had a strong negative impact on the future of NBTs in this continent. Following a request of the European Commission (EC), the European Food Safety Authority (EFSA) evaluated the documentation submitted by several Member States in support of their request for the prohibition of the placing on the market of the GM maize MON 810 for cultivation according to Article 18 of Directive 2002/53/EC. Hungary (in 2005), France (2008), Germany (2009), Italy and Poland (2013), Greece and Bulgaria (2014) and Northern Ireland (2015), etc. requested the EC to adopt emergency measures to prohibit the cultivation of the GM maize MON 810. In 2015 several countries such as France, Germany, Austria, Greece, Hungary, the Netherlands, Latvia, Lithuania, Luxembourg, Bulgaria, Poland, Denmark, Malta, Slovenia, Italy and Croatia have chosen a total ban while Wallonia, the French-speaking region of Belgium has opted out, as well as Scotland, Wales and Northern Ireland (according to the EU Directive 2015/412). Based on EFSA opinions, around 80 events currently have approval for import into the EU (mainly herbicide tolerance) in 2020. How the genome editing policy in Europe is obstructing the development of new traits and is negatively influencing governance decisions and trade worldwide is described by Schiemann et al. (2020). Urnov, Ronald and Caroll (2018) come to conclusions that despite the Curia decision regulatory corrections are still possible, they say: “The CJEU ruling does not explicitly ban gene-edited crops. Instead, it categorizes them with transgenic plants and subjects them to such extensive risk evaluation that the cost of gaining approval could be borne by only the largest corporations. From a scientific perspective, this is, in plain terms, nonsensical: as explained above, thousands of crops produced with radiation carry a wealth of small genetic changes and are deemed safe. Why would a crop in which just one such change has been introduced by genome editing be regulated differently?” Overregulation is particularly observed in Europe and a new regulation based on the product rather than on process is required (Ricroch, Ammann & Kuntz 2016). As plants modified by the NBTs are considered GMOs, it can be predicted that there will be fewer trials using these techniques in a near future, as was the case with transgenesis as early as 2006. The creativity of European researchers (breeders in public and private laboratories) is in danger of being hindered. This creativity could have been at the service of climate smart agriculture. Only UK, which is outside the European framework, retains the freedom to carry out research with the latest genetic and genomic tools. Europe will import agricultural products modified by transgenesis and NBTs from China and USA which are the leaders in the world.
ACKNOWLEDGEMENTS
The contribution of Oumaima Ouni, Victor Pallares and Bleuenn Rault to early stages of this work is gratefully acknowledged. Dr Marcel Kuntz is acknowledged for his valuable comments.
References
EuropaBio (2020). Genome-Edited Products and Projects: Resources and Examples. https://www.europabio.org/priority/genome-editing
Herrera-Estrella L., Depicker A., Van Montagu M. et al. Expression of chimaeric genes transferred into plant cells using a Ti-plasmid-derived vector. Nature 303, 209–213 (1983). https://doi.org/10.1038/303209a0).
Kuntz M. & A. Berezow (2017). A. Timidity and a hostility to competition have left Europe a scientific wasteland. The Telegraph, 5 September
Pfeiffer M., F. Quétier & Ricroch A. (2018). Genome editing in plant breeding. In: special issue ‘Transgenic plants and beyond’. Advances in Botanical Research (M. Kuntz, Editor). 86, 245-286 https://www.sciencedirect.com/science/bookseries/00652296
Qaim M. (2020). Role of new plant breeding technologies for food security and sustainable agricultural development. Applied Economic Perspectives and Policy. https://doi.org/10.1002/aepp.13044
Ricroch A. (2019). Keynote at the OECD Conference, 28th May 2018. Global Developments of Genome Editing in Agriculture. Transgenic Research, 133 DOI :10.1007/s11248-019-00133-6.
Ricroch A., Boisron A. & M. Kuntz (2015). Looking back at safety assessment of GM food/feed: an exhaustive review of 90-day animal feeding studies. International journal of biotechnology, special issue “Impacts of Agricultural Biotechnology: Long-Term Evidence”13, 4, 2014. 230-256
Ricroch A., Clairand P., & W. Harwood (2017). Use of CRISPR systems in plant genome editing: towards new opportunities in agriculture. In: special issue. Emerging Topics in Life Emerging Topics in Life Sciences 1 169–182
Ricroch A., Ammann K. & M. Kuntz (2016). Editing EU legislation to fit plant genome editing. EMBO Reports 17, 1365-1369. Sept. 14, 2016 online. Sept. 14, 2016 online
Schiemann J., Robienski J., Schleissing S., Spök A., Sprink T., & R.A. Wilhelm (2020). Plant Genome Editing – Policies and Governance Front. Plant Sci. doi: 10.3389/fpls.2020.00284
Urnov F. D., Ronald P. C. & D. Carroll (2018). A call for science-based review of the European court’s decision on gene-edited crops. Nature Biotechnology, 01 Sep 2018 36(9) 800-802. DOI: 10.1038/nbt.4252
Wang Y., Cheng X., Shan Q. et al. (2014). Simultaneous editing of three homoeoalleles in hexaploid bread wheat confers heritable resistance to powdery mildew. Nature Biotechnology 32,947–951 https://doi.org/10.1038/nbt.2969